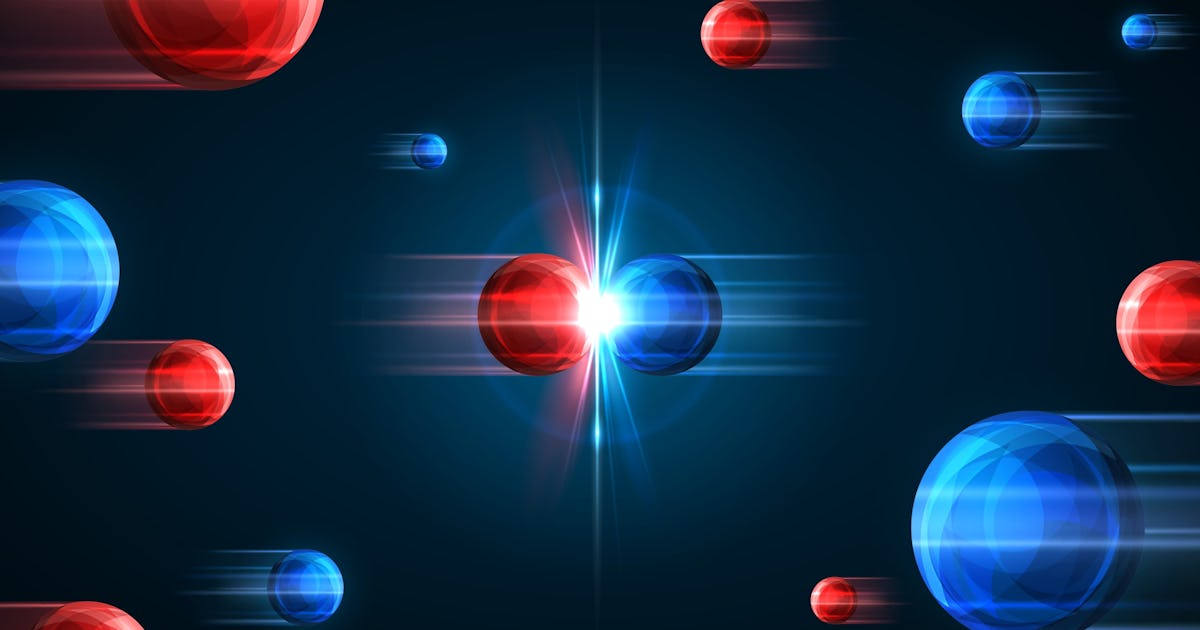
A new surprising result from digging in Fermilab archives could help scientists uncover new laws of physics and solve mysteries such as the nature of dark matter.
In a new study appearing in the April 8 issue of the journal Science, the most precise measurement yet of a key particle linked with the weak force known as the W boson reveals it appears surprisingly heavier than previously thought.
Here’s the background — The weak force is one of the four known fundamental forces of nature — the other three are gravity, electromagnetism, and the strong force. It helps trigger the nuclear reactions that keep the sun burning and also drives the decay of particles. Also known as the weak interaction or the weak nuclear force, it is effective only to a distance of about 10^-17 meters, or roughly 1 percent of the diameter of a typical atomic nucleus. It gets its name from how it is much weaker than both the strong force and electromagnetism, although all three are stronger than gravity.
The weak force can help protons turn into neutrons and vice versa. This helps initiate the nuclear fusion reactions that power stars, and can also lead radioactive nuclei to decay. In addition, most subatomic particles are unstable and decay via the weak force. The existence of the W boson as an agent of the weak force was predicted in the 1960s and first confirmed in 1983 at CERN, the largest particle physics lab in the world.
The W boson is a key building block of the Standard Model, which is currently the best explanation for how all the known elementary particles behave. However, the Standard Model has a number of major shortcomings — for example, it currently cannot explain dark matter, a thus-far invisible substance thought to make up roughly five-sixths of all the matter in the universe. It also doesn’t account for gravity or the excess of matter over antimatter in the universe. Any new discoveries at odds with the Standard Model’s predictions may lead the way to a better model beyond it.
WHAT DID THE SCIENTISTS DO? — The researchers spent nearly a decade examining data from the Tevatron particle accelerator at Fermilab, once the world’s largest particle accelerator. They analyzed huge amounts of data from the now-defunct accelerator generated from 2002 to 2011 regarding about 4 million W boson candidates, around four times as many used in previous Tevatron-based measurements of the particle’s masss.
The Tevatron collided protons and antiprotons at nearly the speed of light, creating flashes of energy that coalesced into particles that in turn decayed into more stable particles. As the debris from these collisions passed through Tevatron’s detectors, scientists could deduce their energy, path, and charge, helping them measure particles such as the W boson.
“We used mature techniques from previous analyses and developed many new analysis techniques,” study co-author Ashutosh Kotwal, a particle physicist at Duke University who initiated and led the W boson mass analyses, tells Inverse. “We implemented new ideas to use our data in novel ways to calibrate our experimental apparatus much more precisely than in the past.”
“We also incorporated new information about the colliding proton’s structure that the particle physics community has collected over the last decade,” he adds. “The combination of a four times larger dataset, more insightful methods and ideas of using our data, and new information about the proton structure allowed us to improve the precision of this measurement substantially.”
WHAT DID THEY FIND? — The new study estimated the W boson had a mass of about 80.433 billion electronvolts, with a precision of 0.01 percent. This is twice as precise as the previous best measurement, and corresponds to measuring the weight of an 800-pound gorilla to within 1.5 ounces.
“The precision of this very delicate and challenging measurement is quite impressive,” Martijn Mulders, an experimental particle physicist at CERN who did not participate in this study, tells Inverse.
However, this new estimate is roughly 76 million electronvolts heavier than what the Standard Model predicts as the W boson’s mass — about 80.357 billion electronvolts. In comparison, the electron’s mass is about 0.5 million electronvolts, whereas the proton’s mass is about 938 million electronvolts.
“The deviation from the Standard Model prediction is very large,” Claudio Campagnari, an experimental particle physicist at the University of California at Santa Barbara who did not take part in this research, tells Inverse. “If the result is confirmed by another experiment, and if a careful re-examination of the theoretical calculation, including the other experimental inputs that go into it, does not find any problem, this would be solid evidence that there is new physics beyond what is encoded into the Standard Model of particles and their interactions.”
WHAT’S NEXT? — Since extraordinary claims require extraordinary evidence, additional experiments are needed to confirm these new findings. “The big question will be, ‘Can anyone find anything wrong?'” Mulders says.
Particle accelerators that might carry out such work include the Large Hadron Collider at CERN, as well as proposed colliders such as the International Linear Collider in Japan, the Compact Linear Collider, the Future Circular Collider at CERN, and the Circular Electron Positron Collider in China. The Future Circular Collider offers the best prospects for better W boson mass measurements, with a projected sensitivity of 7 parts per million, more than 10 times better than the current best measurement, Mulders and Campagnari wrote in a commentary on the new study.
These measurements of the W boson’s mass do require a very precisely calibrated detector, and given how the calibration procedure is quite complicated, something might have gone wrong there with Tevatron’s results, potentially explaining this deviation. “On the other hand, the people that carried out this work are some of the world’s experts in these type of measurements,” Campagnari says.
If this result is accurate, previous research might not have measured the W boson’s mass properly for a number of reasons. Perhaps the particle accelerators used in prior work lacked the power to generate new massive particles whose interactions with the W boson influence its mass, or perhaps these other particles were very difficult to measure by other detectors for other reasons, Mulders says.
“If confirmed, the difference between the measured value and the Standard Model calculation of the W boson mass would have to be due to a new mechanism in nature, a new fundamental principle we do not know about,” Kotwal says. “This could manifest as a new particle or subatomic interaction and be discovered in running and future experiments.”